4 Chapter 4 Weathering and Erosion
R. Adam Dastrup
Weathering
Weathering is what takes place when a body of rock is exposed to the “weather” — in other words, to the forces and conditions that exist at Earth’s surface. Except for volcanic rocks and some sedimentary rocks, most rocks are formed at some depth within the crust. There they experience relatively constant temperature, high pressure, no contact with the atmosphere, and little or no moving water. Once a rock is exposed at the surface, which is what happens when the overlying rock is eroded, conditions change dramatically. Temperatures vary widely, there is much less pressure, oxygen and other gases are plentiful, and in most climates, water is abundant.

Weathering includes two main processes that are entirely different. One is the mechanical breakdown of rock into smaller fragments, and the other is the chemical change of the minerals within the rock to forms that are stable in the surface environment. Mechanical weathering provides fresh surfaces for attack by chemical processes, and chemical weathering weakens the rock so that it is more susceptible to mechanical weathering. Together, these processes create two significant products, one being the sedimentary clasts and ions in solution that can eventually become sedimentary rock, and the other being the soil that is necessary for our existence on Earth.
4.1 Mechanical Weathering
Intrusive igneous rocks form at depths of several hundreds of meters to several tens of kilometers. Sediments are turned into sedimentary rocks only when other sediments bury them to depths more than several hundreds of meters. Most metamorphic rocks are formed at depths of kilometers to tens of kilometers. Weathering cannot even begin until these rocks are uplifted through various processes of mountain building — most of which are related to plate tectonics — and the overlying material has been eroded, and the rock is exposed as an outcrop.
The critical agents of mechanical weathering are:
- The decrease in pressure that results from the removal of overlying rock
- Freezing and thawing of water in cracks in the rock
- Formation of salt crystals within the rock
- Cracking from plant roots and exposure by burrowing animals
When a mass of rock is exposed by weathering and removal of the overlying rock, there is a decrease in the confining pressure on the rock, and the rock expands. This unloading promotes cracking of the rock, known as exfoliation.
Granitic rock tends to exfoliate parallel to the exposed surface because the rock is typically homogenous, and it does not have predetermined planes along which it must fracture. Sedimentary and metamorphic rocks, on the other hand, tend to exfoliate along predetermined planes.
Frost wedging, also called ice wedging, is the process by which water seeps into cracks in a rock, expands on freezing, and thus enlarges the cracks. The effectiveness of frost wedging is related to the frequency of freezing and thawing. Frost wedging is most effective in mountainous climates. In warm areas where freezing is infrequent, in very cold areas where thawing is infrequent, or in arid areas, where there is little water to seep into cracks, the role of frost wedging is limited.
In many mountainous regions, the transition between freezing nighttime temperatures and thawing daytime temperatures is frequent — tens to hundreds of times a year. Even in warm coastal areas, freezing and thawing transitions are common at higher elevations. A common feature in areas of active frost wedging is a talus slope — a fan-shaped deposit of fragments removed by frost wedging from the steep rocky slopes above.

A related process, frost heaving, takes place within unconsolidated materials on gentle slopes. In this case, water in the soil freezes and expands, pushing the overlying material up. Frost heaving is responsible for winter damage to roads all over North America.
When saltwater seeps into rocks and then evaporates on a hot sunny day, salt crystals grow within cracks and pores in the rock. The growth of these crystals exerts pressure on the rock and can push grains apart, causing the rock to weaken and break. Salt weathering can also occur away from the coast because most environments have some salt in them.
The effects of plants and animals are significant in mechanical weathering. Roots can force their way into even the tiniest cracks, and then they exert tremendous pressure on the rocks as they grow, widening the cracks and breaking the rock. Although animals do generally not burrow through solid rock, they can excavate and remove huge volumes of soil, and thus expose the rock to weathering by other mechanisms.
Mechanical weathering is greatly facilitated by erosion, which is the removal of weathering products, allowing for the exposure of more rock for weathering. On the steep rock faces at the top of the cliff, rock fragments have been broken off by ice wedging, and then removed by gravity. This is a form of mass wasting. Other essential agents of erosion that also have the effect of removing the products of weathering include water in streams, ice in glaciers, and waves on the coasts.
4.2 Chemical Weathering
Chemical weathering results from chemical changes to minerals that become unstable when they are exposed to surface conditions. The kinds of changes that take place are highly specific to the mineral and the environmental conditions. Some minerals, like quartz, are virtually unaffected by chemical weathering, while others, like feldspar, are easily altered. In general, the degree of chemical weathering is most significant in warm and wet climates and least in cold and dry climates. The important characteristics of surface conditions that lead to chemical weathering are the presence of water (in the air and on the ground surface), the abundance of oxygen, and the presence of carbon dioxide, which produces weak carbonic acid when combined with water.
The Products of Weathering and Erosion
The products of weathering and erosion are the unconsolidated materials that we find around us on slopes, beneath glaciers, in stream valleys, on beaches, and in deserts. The nature of these materials — their composition, size, the degree of sorting, and degree of rounding — is determined by the type of rock that is being weathered, the nature of the weathering, the erosion, and transportation processes, and the climate.
The produces created from weathering range widely in size and shape depending on the processes involved. If and when deposits like these are turned into sedimentary rocks, the textures of those rocks will vary significantly. Importantly, when we describe sedimentary rocks that formed millions of years in the past, we can use those properties to make inferences about the conditions that existed during their formation.
4.3 Weathering and the Formation of Soil
Weathering is a key part of the process of soil formation, and soil is critical to our existence on Earth. Many people refer to any loose material on Earth’s surface as soil, but to geologists (and geology students) soil is the material that includes organic matter, lies within the top few tens of centimeters of the surface, and is vital in sustaining plant growth.
Soil is a complex mixture of minerals (approximately 45 percent), organic matter (approximately 5 percent), and empty space (approximately 50 percent, filled to varying degrees with air and water). The mineral content of soils is variable, but is dominated by clay minerals and quartz, along with minor amounts of feldspar and small fragments of rock. The types of weathering that take place within a region have a significant influence on soil composition and texture. For example, in a warm climate, where chemical weathering dominates, soils tend to be more abundant in clay. Soil scientists describe soil texture in terms of the relative proportions of sand, silt, and clay. The sand and silt components in this diagram are dominated by quartz, with lesser amounts of feldspar and rock fragments, while the clay component is dominated by the clay minerals.
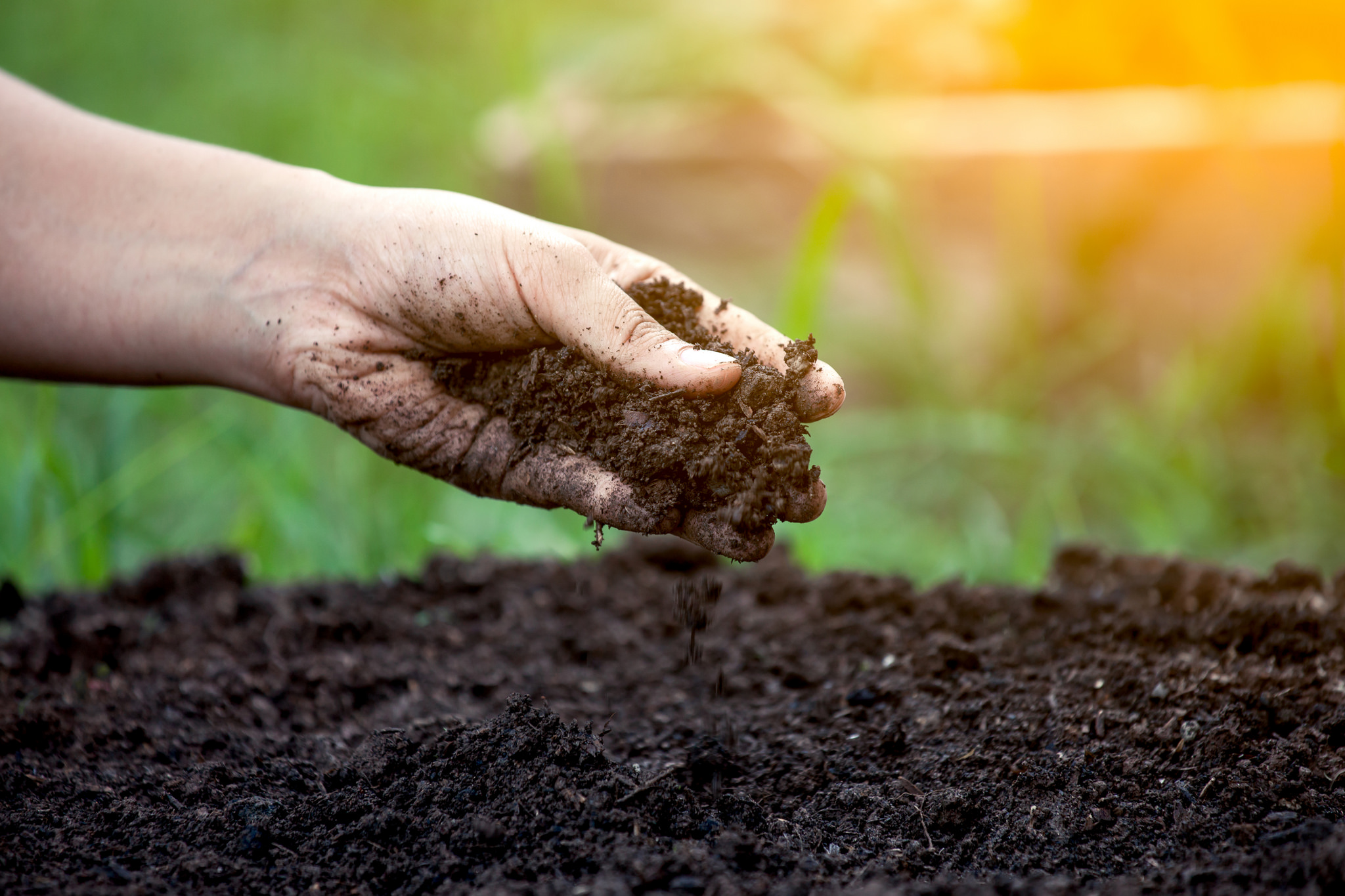
Soil forms through accumulation and decay of organic matter and the mechanical and chemical weathering processes described above. The factors that affect the nature of soil and the rate of its formation include climate (especially average temperature and precipitation amounts, and the following types of vegetation), the type of parent material, the slope of the surface, and the amount of time available.
Water erosion is accentuated on sloped surfaces because fast-flowing water has greater eroding power than still water. Raindrops can disaggregate exposed soil particles, putting the finer material (e.g., clays) into suspension in the water. Sheetwash, unchannelled flow across a surface carries suspended material away, and channels erode right through the soil layer, removing both fine and coarse material.
Wind erosion is exacerbated by the removal of trees that act as windbreaks and by agricultural practices that leave bare soil exposed.
Tillage is also a factor in soil erosion, especially on slopes, because each time the soil is lifted by a cultivator, it is moved a few centimeters down the slope.
4.4 Mass Wasting
Mass wasting, which is synonymous with “slope failure,” is the failure and downslope movement of rock or unconsolidated materials in response to gravity. The term “landslide” is almost synonymous with mass wasting, but not quite because some people reserve “landslide” for relatively rapid slope failures, while others do not. Other than the video below, this textbook will avoid using the term “landslide.”
Factors That Control Slope Stability
Mass wasting happens because tectonic processes have created uplift. Erosion, driven by gravity, is the inevitable response to that uplift, and various types of erosion, including mass wasting, have created slopes in the uplifted regions. Slope stability is ultimately determined by two factors: the angle of the slope and the strength of the materials on it.
A block of rock is typically situated on a rock slope that is being pulled toward Earth’s center (vertically down) by gravity. The vertical gravitational force can be split into two components relative to the slope: one pushing the block down the slope (the shear force), and the other pushing into the slope (the normal force). The shear force, which wants to push the block down the slope, has to overcome the strength of the connection between the block and the slope, which may be quite weak if the block has split away from the main body of rock, or may be very strong if the block is still a part of the rock. If the shear strength is greater than the shear force, the block should not move. But if the shear force becomes stronger than the shear strength, the block of rock will slide down the slope.
As already noted, slopes are created by uplift followed by erosion. In areas with relatively recent uplift, slopes tend to be quite steep. This is especially true where glaciation has taken place because glaciers in mountainous terrain create steep-sided valleys. In areas without recent uplift, slopes are less steep because hundreds of millions of years of erosion (including mass wasting) have made them that way. However, as we will see, some mass wasting can happen even on relatively gentle slopes.
The strength of the materials on slopes can vary widely. Solid rocks tend to be strong, but there is an extensive range of rock strength. If we consider just the strength of the rocks, and ignore issues like fracturing and layering, then most crystalline rocks, like granite, basalt, or gneiss, are very strong, while some metamorphic rocks, like schist, are moderately strong. Sedimentary rocks have variable strength. Dolostone and some limestone are strong, most sandstone and conglomerate are moderately strong, and some sandstone and all mudstones are quite weak.
Fractures, metamorphic foliation, or bedding can significantly reduce the strength of a body of rock, and in the context of mass wasting, this is most critical if the planes of weakness are parallel to the slope and least critical if they are perpendicular to the slope.
Internal variations in the composition and structure of rocks can significantly affect their strength. Schist, for example, may have layers that are rich in sheet silicates (mica or chlorite) and these will tend to be weaker than other layers. Some minerals tend to be more susceptible to weathering than others, and the weathered products are commonly quite weak (e.g., the clay formed from feldspar).
Unconsolidated sediments are generally weaker than sedimentary rocks because they are not cemented and, in most cases, have not been significantly compressed by overlying materials. This binding property of sediment is sometimes referred to as cohesion. Sand and silt tend to be particularly weak, clay is generally a little stronger, and sand mixed with clay can be stronger still. Finer deposits are relatively strong (they maintain a steep slope), while the overlying sand is relatively weak, and has a shallower slope that has recently failed. Glacial till, typically a mixture of clay, silt, sand, gravel, and larger clasts, forms and is compressed beneath tens to thousands of meters of glacial ice so it can be as strong as some sedimentary rock.
Apart from the type of material on a slope, the amount of water that the material contains is the most important factor controlling its strength. This is especially true for unconsolidated materials, but it also applies to bodies of rock. Granular sediments, like the sand at Point Grey, have lots of spaces between the grains. Those spaces may be completely dry (filled only with air); or moist (often meaning that some spaces are water filled, some grains have a film of water around them, and small amounts of water are present where grains are touching each other); or completely saturated. Unconsolidated sediments tend to be strongest when they are moist because the small amounts of water at the grain boundaries hold the grains together with surface tension. Dry sediments are held together only by the friction between grains, and if they are well sorted or well rounded, or both, that cohesion is weak. Saturated sediments tend to be the weakest of all because the large amount of water pushes the grains apart, reducing the mount friction between grains. This is especially true if the water is under pressure.
Water will also reduce the strength of solid rock, especially if it has fractures, bedding planes, or clay-bearing zones. This effect is even more significant when the water is under pressure, which is why holes are drilled into rocks on road cuts to relieve this pressure.
Moreover, finally, water can significantly increase the mass of the material on a slope, which increases the gravitational force pushing it down. A body of sediment that has 25% porosity and is saturated with water weighs approximately 13% more than it does when it is completely dry, so the gravitational shear force is also 13% higher.
4.5 Classification of Mass Wasting
It is important to classify slope failures so that we can understand what causes them and learn how to mitigate their effects. The three criteria used to describe slope failures are:
- The type of material that failed (typically either bedrock or unconsolidated sediment)
- The mechanism of the failure (how the material moved)
- The rate at which it moved
The type of motion is the essential characteristic of slope failure, and there are three different types of motion:
- If the material drops through the air, vertically or nearly vertically, it is known as a fall.
- If the material moves as a mass along a sloping surface (without internal motion within the mass), it is a slide.
- If the material has internal motion, like a fluid, it is a flow.
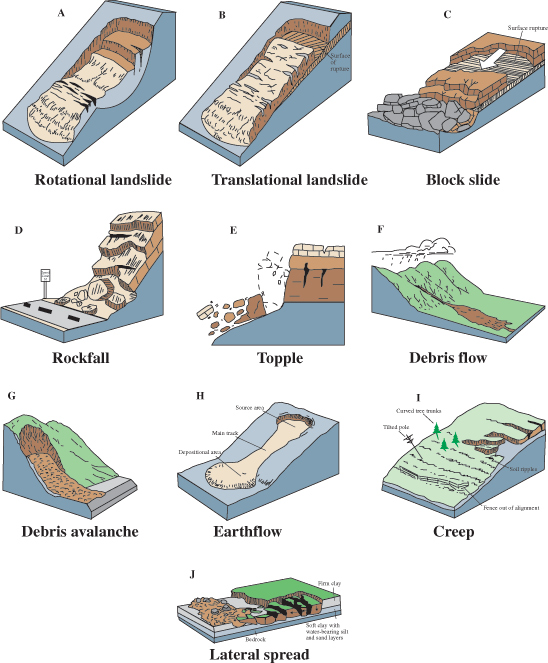
Unfortunately, it is not typically that simple. Many slope failures involve two of these types of motion, some involve all three, and in many cases, it is not easy to tell how the material moved.
Rock Fall
Rock fragments can break off relatively easily from steep bedrock slopes, most commonly due to frost-wedging in areas where there are many freeze-thaw cycles per year. When hiking along a steep mountain trail on a cool morning, one might have heard the occasional fall of rock fragments onto a talus slope. This happens because the water between cracks freezes and expands overnight, and then when that same water thaws in the morning sun, the fragments that had been pushed beyond their limit by the ice fall to the slope below.

Rock Slide
A rock slide is the sliding motion of rock along a sloping surface. In most cases, the movement is parallel to a fracture, bedding, or metamorphic foliation plane, and it can range from very slow to moderately fast. The word sackung describes the very slow motion of a block of rock (mm/y to cm/y) on a slope.
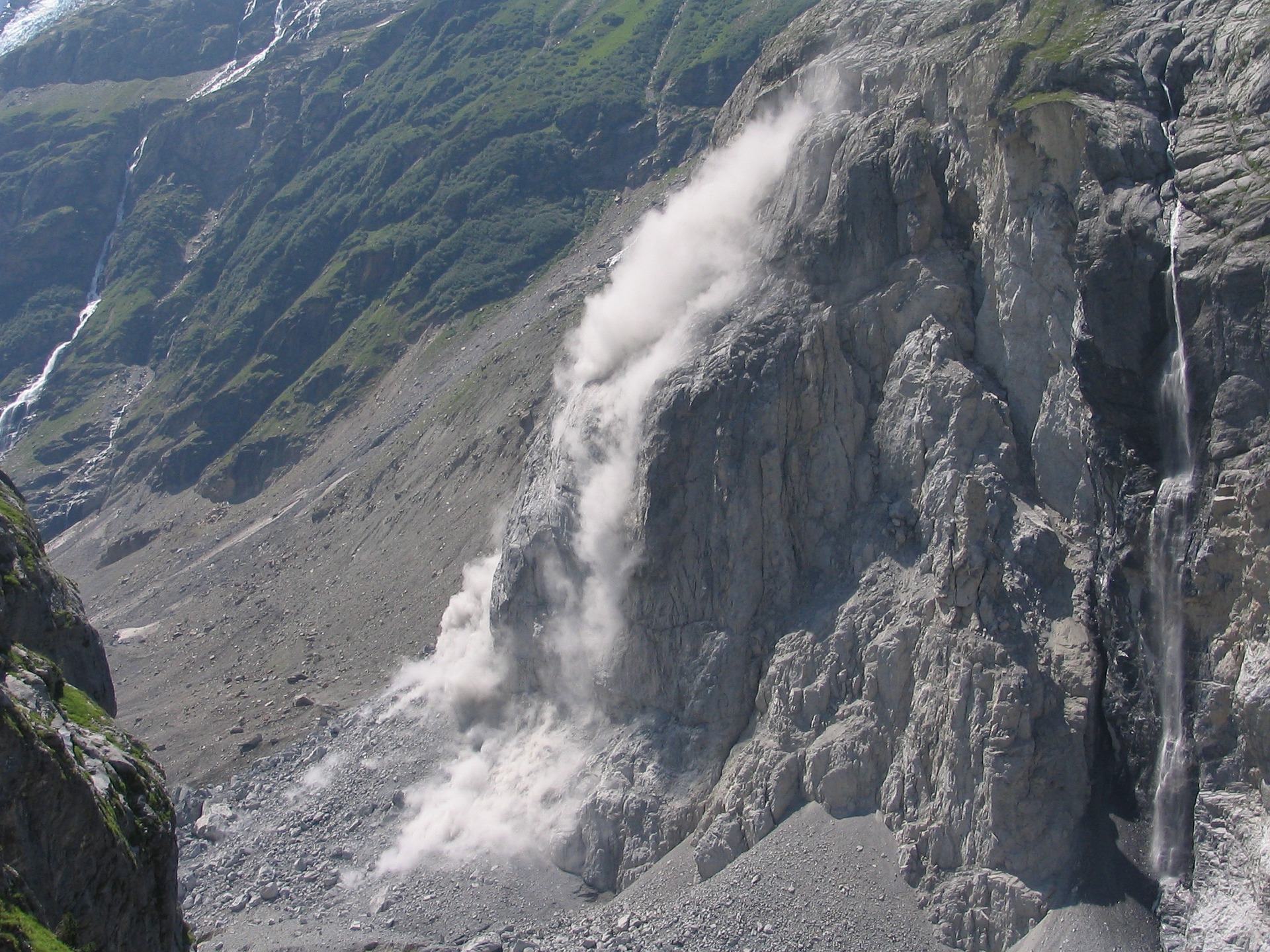
Rock Avalanche
If a rock slides and then starts moving quickly (m/s), the rock is likely to break into many small pieces, and at that point it turns into a rock avalanche, in which the large and small fragments of rock move in a fluid manner supported by a cushion of air within and beneath the moving mass.

Creep or Solifluction
The very slow, millimeters per year to centimeters per year, movement of soil or other unconsolidated material on a slope is known as creep. Creep, which generally only affects the upper several centimeters of loose material, is typically a very slow flow, but in some cases, sliding may take place. Creep can be facilitated by freezing and thawing because particles are lifted perpendicular to the surface by the growth of ice crystals within the soil, and then let down vertically by gravity when the ice melts. The same effect can be produced by frequent wetting and drying of the soil. In cold environments, solifluction is a more intense form of freeze-thaw-triggered creep.
Creep is most noticeable on moderate-to-steep slopes where trees, fence posts, or grave markers are consistently leaning in a downhill direction. In the case of trees, they try to correct their lean by growing upright, and this leads to a curved lower trunk known as a “pistol butt.”

Slump
Slump is a type of slide (movement as a mass) that takes place within thick unconsolidated deposits (typically thicker than 10 m). Slumps involve movement along one or more curved failure surfaces, with downward motion near the top and outward motion toward the bottom. They are typically caused by an excess of water within these materials on a steep slope.
Mudflows and Debris Flows
When a mass of sediment becomes completely saturated with water, the mass loses strength, to the extent that the grains are pushed apart, and it will flow, even on a gentle slope. This can happen during rapid spring snowmelt or heavy rains, and is also relatively common during volcanic eruptions because of the rapid melting of snow and ice. (A mudflow or debris flow on a volcano or during a volcanic eruption is a lahar.) If the material involved is primarily sand-sized or smaller, it is known as a mudflow.
If the material involved is gravel-sized or larger, it is known as a debris flow. Because it takes more gravitational energy to move larger particles, a debris flow typically forms in an area with steeper slopes and more water than does a mudflow. In many cases, a debris flow takes place within a steep stream channel, and is triggered by the collapse of bank material into the stream. This creates a temporary dam, and then a significant flow of water and debris when the dam breaks.
The United States Geologic Survey and the Utah Geologic Survey are excellent sources for more information regarding mass wasting.
4.6 Erosion
Erosion is a mechanical process, usually driven by water, gravity, wind, or ice that removes sediment from the place of weathering. Liquid water is the principal agent of erosion. Erosion resistance is essential in the creation of distinctive geological features. This is well demonstrated in the cliffs of the Grand Canyon. The cliffs are made of rock left standing after less resistant materials have weathered and eroded. Rocks with different levels of erosion resistant also create the unique-looking features called hoodoos in Bryce Canyon National Park and Goblin Valley State Park in Utah.
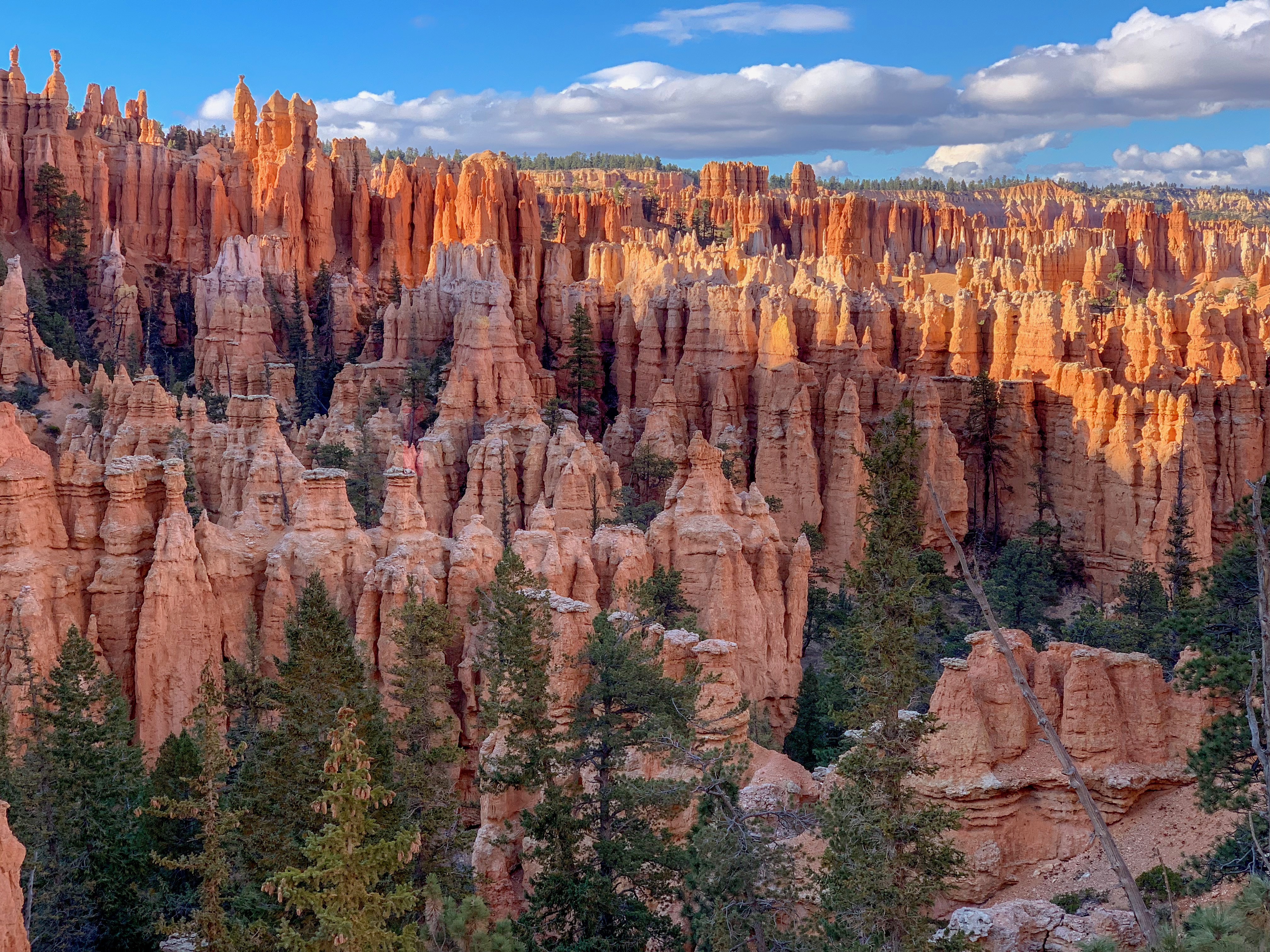
Erosion by Streams
Streams, any running water from a rivulet to a raging river, complete the hydrologic cycle by returning precipitation that falls on land to the oceans. Some of this water moves over the surface and some moves through the ground as groundwater. Flowing water does the work of both erosion and deposition.
EROSION BY STREAMS
Flowing streams pick up and transport weathered materials by eroding sediments from their banks. Streams also carry ions and ionic compounds that dissolve easily in the water. Sediments are carried as the following loads: dissolved, suspended, and bed. A dissolved load is composed of ions in solution. These ions are usually carried in the water all the way to the ocean.
Sediments carried as solids as the stream flows are called a suspended load. The size of particles that can be carried within a load is determined by the stream’s velocity. Faster streams can carry larger particles. Streams that carry larger particles have greater competence. Streams with a steep gradient (slope) have a faster velocity and greater competence.

Particles that are too large to be carried as suspended loads are bumped and pushed along the stream bed, called bed load. Bed load sediments do not move continuously, but rather in intermittent movements, called saltation. Streams with high velocities and steep gradients do a great deal of down cutting into the stream bed, which is primarily accomplished by movement of particles that make up the bed load.
STAGES OF STREAMS
As a stream flows from higher elevations, like in the mountains, towards lower elevations, like the ocean, the work of the stream changes. At a stream’s headwaters, often high in the mountains, gradients are steep. The stream moves fast and does lots of work eroding the stream bed.
As a stream moves into lower areas, the gradient is not as steep. Now the stream does more work eroding the edges of its banks. Many streams develop curves in their channels called meanders. As streams move onto flatter ground, the stream erodes the outer edges of its banks to carve a floodplain, which is a flat level area surrounding the stream channel.
The base level is where a stream meets a large body of standing water, usually the ocean, but sometimes a lake or pond. Streams work to down cut in their stream beds until they reach base level. The higher the elevation, the farther the stream is from where it will reach the base level and the more cutting it has to do.
As a stream gets closer to the base level, its gradient lowers, and it deposits more material than it erodes. On flatter ground, streams deposit material on the inside of meanders. A stream’s floodplain is much broader and shallower than the stream’s channel. When a stream flows onto its floodplain, its velocity slows, and it deposits much of its load. These sediments are rich in nutrients and make excellent farmland.

A stream at flood stage carries lots of sediments. When its gradient decreases, the stream overflows its banks and broadens its channel. The decrease in gradient causes the stream to deposit its sediments, the largest first. These large sediments build a higher area around the edges of the stream channel, creating natural levees.

When a river enters standing water, its velocity slows to a stop. The stream moves back and forth across the region and drops its sediments in a wide triangular-shaped deposit called a delta. If a stream falls down a steep slope onto a broad flat valley, an alluvial fan develops. Alluvial fans generally form in arid regions.

Ground Water Erosion
Groundwater is a strong erosional force, as it works to dissolve away solid rock. Carbonic acid is especially good at dissolving the rock limestone. Over many years, groundwater travels along small cracks. The water dissolves and carries away the solid rock gradually enlarging the cracks, eventually forming a cave. The video below is drone footage of the world’s largest cave, Hang Son Doong, in Vietnam.
Groundwater carries the dissolved minerals in solution. The minerals may then be deposited, for example, as stalagmites (grows from the top) or stalactites (grows from the bottom). If a stalactite and stalagmite join together, they form a column. One of the wonders of visiting a cave is to witness the beauty of these fantastic and strangely captivating structures. Caves also produce a beautiful rock, formed from calcium carbonate, travertine. Groundwater saturated with calcium carbonate precipitates as the mineral calcite or aragonite. Mineral springs that produce travertine can be hot, warm or even cold.
If the roof of a cave collapses, a sinkhole could form. Some sinkholes are large enough to swallow up a home or several homes in a neighborhood.
Wave Action and Erosion
Waves are essential for building up and breaking down shorelines. Waves transport sand onto and off of beaches, transport sand along beaches, carves structures along the shore. The most massive waves form when the wind is very strong, blows steadily for a long time, and blows over a long distance.

The wind could be strong, but if it gusts for just a short time, large waves will not form. Wave energy does the work of erosion at the shore. Waves approach the shore at some angle, so the inshore part of the wave reaches shallow water sooner than the part that is further out. The shallow part of the wave ‘feels’ the bottom first. This slows down the inshore part of the wave and makes the wave ‘bend.’ This bending is called refraction.
Wave refraction either concentrates wave energy or disperses it. In quiet water areas, such as bays, wave energy is dispersed, so sand is deposited. Areas that stick out into the water are eroded by the intense wave energy that concentrates its power on the wave-cut cliff.
A wave-cut platform is the level area formed by wave erosion as the waves undercut a cliff. An arch is produced when waves erode through a cliff. When a sea arch collapses, the isolated towers of rocks that remain are known as sea stacks.
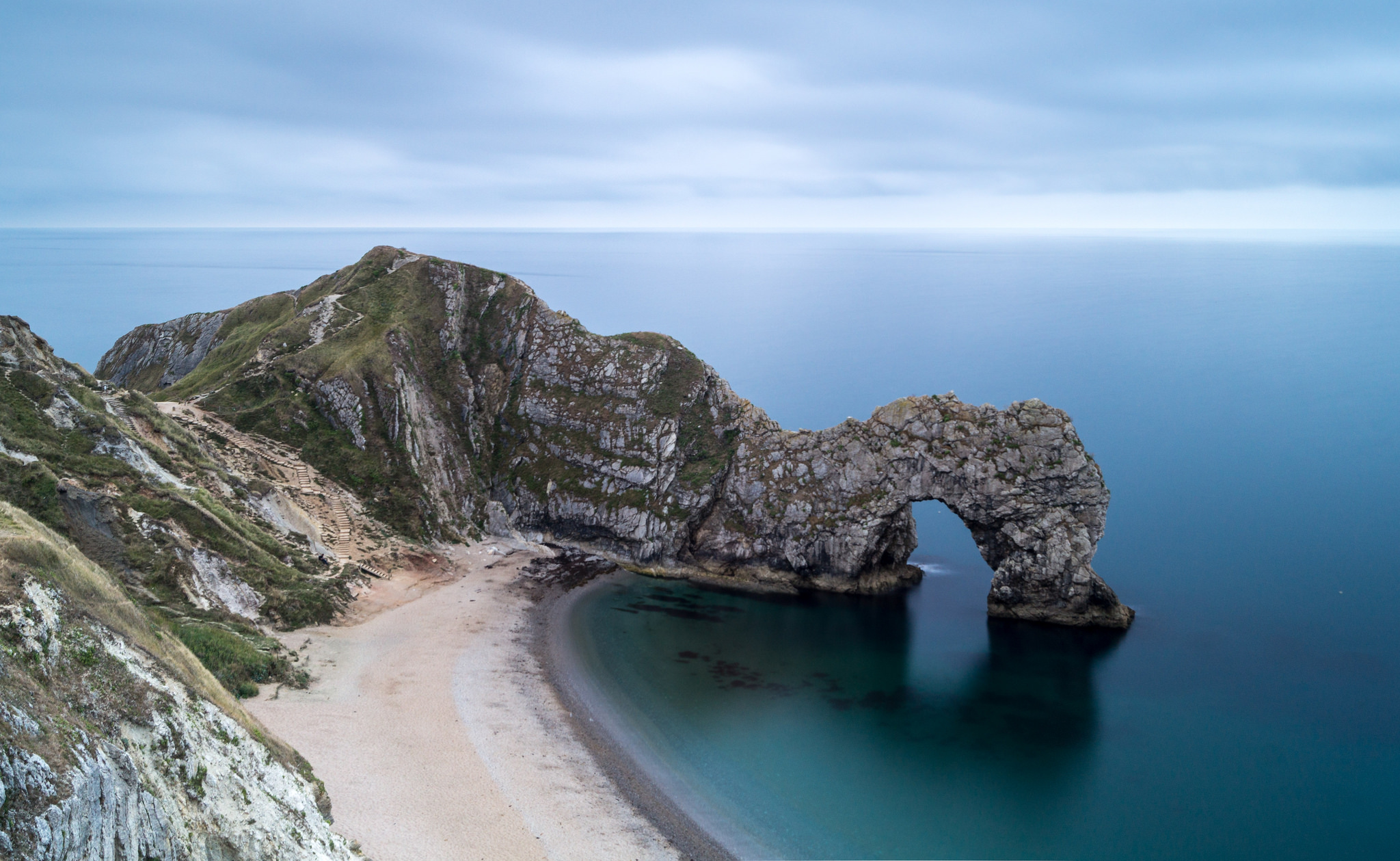
WAVE DEPOSITION
Rivers carry sediments from the land to the sea. If wave action is high, a delta will not form. Waves will spread the sediments along the coastline to create a beach. Waves also erode sediments from cliffs and shorelines and transport them onto beaches.
Beaches can be made of mineral grains, like quartz, rock fragments, and also pieces of shell or coral. Waves continually move sand along the shore and move sand from the beaches on shore to bars of sand offshore as the season’s change. In the summer, waves have lower energy, so they bring sand up onto the beach. In the winter, higher energy waves bring the sand back offshore.
Some features form by wave-deposited sand. These features include barrier islands and spits. A spit is sand connected to the land and extending into the water. A spit may hook to form a tombolo. Shores that are relatively flat and gently sloping may be lined with long narrow barrier islands. Most barrier islands are a few kilometers wide and tens of kilometers long.
In its natural state, a barrier island acts as the first line of defense against storms such as hurricanes. When barrier islands are urbanized, hurricanes damage houses and businesses rather than vegetated sandy areas in which sand can move. A large hurricane brings massive problems to the urbanized area.
PROTECTING SHORELINES
Intact shore areas protect inland areas from storms that come off the ocean. Where the natural landscape is altered, or the amount of development does damage from a storm too costly to consider, people use several types of structures to attempt to slow down wave erosion. A groin is a long narrow pile of rocks built perpendicular to the shoreline to keep sand at that beach. A breakwater is a structure built in the water parallel to the shore in order to protect the shore from strong incoming waves. A seawall is also parallel to the shore, but it is built onshore.

People do not always want to choose safe building practices, and instead choose to build a beach house right on the beach. Protecting development from wave erosion is difficult and expensive, and it does not always work. The northeastern coast of Japan was protected by anti-tsunami seawalls, yet waves from the 2011 tsunami that resulted from the Tohoku earthquake washed over the top of some seawalls and caused others to collapse. Japan is now planning to build even higher seawalls to prepare for any future (and inevitable) tsunami.
4.7 Transport of Particles by Wind
The power of wind to erode depends on particle size, wind strength, and whether the particles can be picked up. Wind is a more important erosional force in arid than humid regions. Wind transports small particles, such as silt and clay, over great distances, even halfway across a continent or an entire ocean basin. Particles may be suspended for days. Wind more easily picks up particles on the ground that has been disturbed, such as a construction site or a dune.
wind erosion
Wind is a stronger erosional force in arid regions than it is in humid regions. In humid areas, water and vegetation bind the soil, so it is harder to pick up. In arid regions, small particles are selectively picked up and transported. As they are removed, the ground surface gets lower and rockier, causing deflation. What is left is desert pavement, a surface covered by gravel-sized particles that are not easily moved by wind.
Particles moved by wind do the work of abrasion. As a grain strikes another grain or surface, it erodes that surface. Abrasion by wind may polish natural or human-made surfaces, such as buildings. Stones that have become polished and faceted due to abrasion by sand particles are called ventifacts.
Exposed rocks in desert areas often develop a dark brown to black coating called desert varnish. Wind transports clay-sized particles that chemically react with other substances at high temperatures. The coating is formed of iron and manganese oxides. Often petroglyphs are carved into the desert varnish by earlier civilizations in arid regions.

4.8 Glacial Influence on Erosion
Formation and Movement of Glaciers
Glaciers cover about 10 percent of the land surface near Earth’s poles, and they are also found in high mountains. During the Ice Ages, glaciers covered as much as 30 percent of Earth. Around 600 to 800 million years ago, geologists think that almost all of the Earth was covered in snow and ice, called the Snowball Theory. Scientists use the evidence of erosion and deposition left by glaciers to do a kind of detective work to figure out where the ice once was and where it came from.
Glaciers are solid ice that moves exceptionally slowly along the land surface. They erode and shape the underlying rocks. Glaciers also deposit sediments in characteristic landforms. The two types of glaciers are: continental and alpine. Continental glaciers are large ice sheets that cover relatively flat ground. These glaciers flow outward from where the most considerable amount of snow and ice accumulate. Alpine or valley glaciers flow downhill through mountains along existing valleys.
GLACIAL EROSION
Glaciers erode the underlying rock by abrasion and plucking. Glacial meltwater seeps into cracks of the underlying rock, the water freezes and pushes pieces of rock outward. The rock is then plucked out and carried away by the flowing ice of the moving glacier. With the weight of the ice over them, these rocks can scratch deeply into the underlying bedrock making long, parallel grooves in the bedrock, called glacial striations.
Mountain glaciers leave behind unique erosional features. When a glacier cuts through a ‘V’ shaped river valley, the glacier pucks rocks from the sides and bottom. This widens the valley and steepens the walls, making a ‘U’ shaped valley.
Smaller tributary glaciers, like tributary streams, flow into the main glacier in their own shallower ‘U’ shaped valleys. A hanging valley forms where the main glacier cuts off a tributary glacier and creates a cliff. Streams plunge over the cliff to create waterfalls. Up high on a mountain, where a glacier originates, rocks are pulled away from valley walls.