Asteroid Tracking with a Small Telescope Elizabeth Arhavbarien, Howard Community College |
Abstract
Asteroid observation is vital for the future of space research. Tracking asteroids and determining their positions is key to preventing possible asteroid impacts with Earth and can also lead to future missions dedicated to researching their composition. Since asteroids have not been sampled due to their fast, ellipsoidal trajectories, having accurate position data can decrease the risk of spacecraft missing their asteroid targets. This, in turn, can help researchers discover new information about our solar system and universe. During fall 2021, our team initiated asteroid observation at HCC by performing observations for asteroid astrometry. To make these observations, the team used data from the Minor Planet Center (MPC), which is the primary data site for all astrometry (position) data for comets, asteroids, and other minor planets.
The team hopes to establish an observation program for asteroid tracking at HCC. This would allow HCC to report astrometric observations of minor planets, comets, or irregular natural satellites, all of which would contribute to global knowledge of position data and be stored in the MPC. Contributing to the MPC requires an official observatory code from them, so this became the short-term goal. To accomplish this short-term goal, as many other colleges have done [1], we demonstrated the ability to gather position data on at least eight asteroids from magnitude 14 to 18 by tracking their trajectories using a research telescope. Then, we compared the predicted trajectories of the studied asteroids with the team’s data on those same asteroids. If prediction and observations agreed within 2 arcseconds as stipulated by the MPC, HCC would receive an official observatory code from the MPC and be able to submit observations for less well-known orbits.
In this paper, by method of telescope observation and plate solving, we successfully tracked our target asteroids and obtained position data on these asteroids with an error margin of 1.40 arcseconds. This puts HCC on track to receive an official observation code in the coming months. However, our newly established process is not without its flaws, with the error in Declination (latitude) being double that of the error in Right Ascension (longitude) for a currently unknown reason and with inconsistent measurements in magnitude.
Introduction
Asteroids have been extremely important to astronomers ever since we could look up at the sky and identify “falling stars”. Today, though, they are of even more critical importance due to the numerous satellites orbiting Earth that are used daily for almost all basic needs. Asteroids, if not tracked, could crash into Earth or the satellites around Earth without warning, causing loss of communication at minimum and possibly loss of life at worst, as seen with the Chelyabinsk meteor in 2013.
Observing asteroids allows scientists to determine their orbits, thus allowing scientists to analyze if, when, and where asteroids could impact humanity. These asteroid observations are also pertinent due to the possibility of discovering new objects in our universe and confirming objects already discovered. Asteroids are made from the same substances as the planet, hence by studying asteroids, not only can we better understand our solar system and the origin of life on the planet, but we could also discover beneficial resources buried in the asteroids and mine them for resources. While most of these benefits look to the future, a current benefit of tracking asteroids is for the people who track them. Tracking asteroids benefits the individuals studying them: determining the position of an asteroid, requires knowledge, skills, and abilities in using a telescope and sky imaging software.
The Minor Planet Center (MPC) is a leading authority in the discovery and confirmation of asteroids, which are scientifically known as minor planets. The MPC stores position data gathered by observers from all over the world and computes the orbits of small solar system bodies using the gathered data [2]. The MPC then updates its computations based on new observations. Amateur astronomers play a critical role in the MPC. The International Astronomical Union, which founded and oversees the MPC [3], is an astronomer group. Ten major amateur astronomer groups, including college groups, that submit to the MPC have helped discover 1194821 (and counting) minor planets [2]. We plan to contribute to this international database of asteroid orbital information by using resources from the MPC to utilize HCC’s telescope to observe and track asteroids.
The team plans to establish an observation program for asteroid tracking at HCC. Since contributing to the MPC requires an official observatory code from them, our short-term goal is to obtain this code. To do this, organizations needed to demonstrate the ability to gather position data on at least eight asteroids from magnitude 14 to 18. The team will obtain and compare the MPC’s predicted trajectories of the eight or more asteroids with the acquired data on those same asteroids. If prediction and observations agree within 2 arcseconds, HCC will receive an official observatory code from the MPC and be able to submit observations for less well-known orbits.
Methods
The research consists of collecting images, analyzing the images, and submitting the results to the Minor Planet Center. Our research requires establishing a process at HCC for each of these steps. Throughout the course of our research and planning, we utilized a few specialized pieces of equipment that were used in prior exoplanet photometry research on campus [4]. The set of equipment used is comprised of a 14” PlaneWave telescope, an AstroPhysics 1100GTO equatorial mount and an SBIG STXL-6303E camera (Figure 1). The equatorial mount shown has a motor aligned with rotation axis of the earth to counteract the earth’s rotation making it possible for the telescope to precisely follow the star [4]. MaximDL was used to capture the images remotely, and the image processing and data analysis was carried out using AstroImageJ. The assembled unit is covered with a protective outdoor cover when not in use.
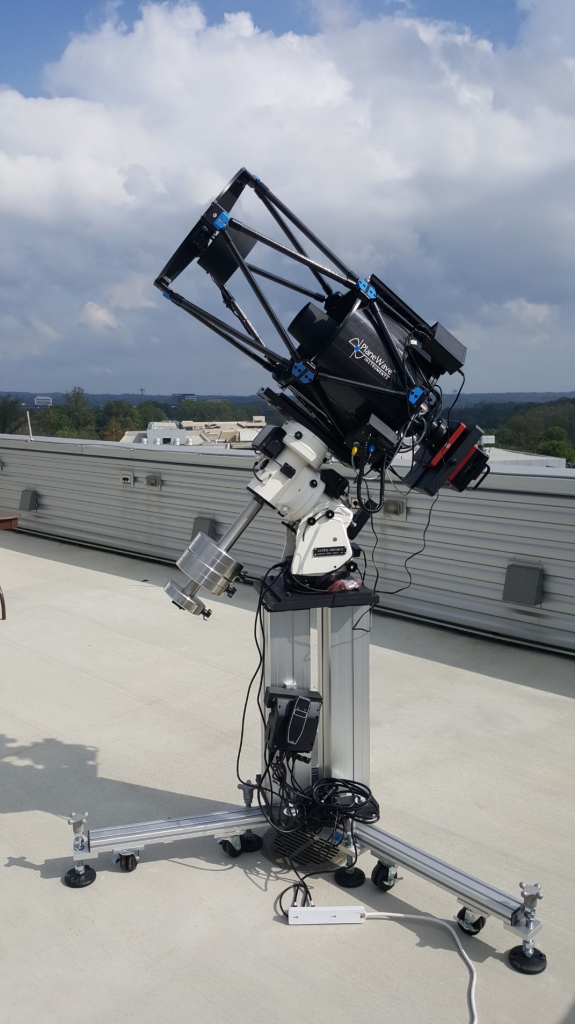
We used a research telescope located on the roof of the SET building to get astronomical images of the sky, and the location and positioning of our target asteroids. Observation occurred over the time span of several hours on multiple days.
To ensure a high signal-to-noise ratio, the targets were observed on clear nights. The images were taken using the camera attached to the telescope which we controlled virtually using Zoom. After this, the telescoping imaging software, AstroImageJ was employed in the alignment and plate-solving of these images, after which position data was acquired from these images such that meaningful information could be gathered and interpreted in terms of right ascension (RA) and declination (Dec). RA and Dec measures the east-west and north-south positions of the stars respectively on the celestial sphere. They are the sky coordinates that correspond to the earth coordinates known as longitude and latitude.
Target Selection
An asteroid catalog on asteroid.lowell.edu was utilized in determining the desired targets. This website allows for the filtering of target asteroids based on magnitude, type, and size. Magnitude refers to the brightness of an asteroid, while type refers to where an asteroid is located in the solar system.
These targets are determined based on the requirements and specifications of the Minor Planet Center (MPC). As such, one of the targets was a near earth asteroid (NEA), the other targets were in the asteroid belt, with the desired magnitude range being between 14-18.
Observation
Images were collected using the 14” Planewave telescope at HCC on clear nights for asteroids in the desired magnitude range and region of the sky. Observations for an individual asteroid were completed multiple times in the same night, at least 30 minutes apart. The software MaximDL was used for image collection and telescope guiding. The website asteroid.lowell.edu provides asteroid predictions from the Minor Planet Center.
It can be verified that the four objects observed are in fact asteroids and not just white spots caused by cosmic rays, as those would be significantly smaller than our asteroids, since they are just a few pixels in size. Additionally, our studied asteroids appeared to follow the same motion as the predicted trajectories from online databases.
During observation, a series of calibration images were obtained after the science images were completed but were not applied as they were not required for positional information, but may be used for magnitude calculations.
Data Reduction
Data Reduction involves the processing of each asteroid image to obtain meaningful information from them. This includes the position, magnitude and the coordinates of the asteroids observed.
After all the necessary images are taken during observation using the telescope and the camera, data analysis and reduction were performed using the imaging software, AstroImageJ.
The process involves aligning the science images such that the target stars in each image are in the same position and coordinate system to account for any imprecise tracking. Aligning of the images allows for better visualization of the path of the asteroid. The next step is to plate solve, which is a process which involves finding the precise coordinates of the center of the science image based on the location of the pattern of stars [5]. When plate solving, we were prompted to select the target star, as well as comparison stars. For this, we arbitrarily selected surrounding stars of the same visibility as the target star. The selected stars are circled red and the option to constrain the search to a particular coordinate in the sky is available. This option was used because it allows for a smaller search region, hence, saving more time.
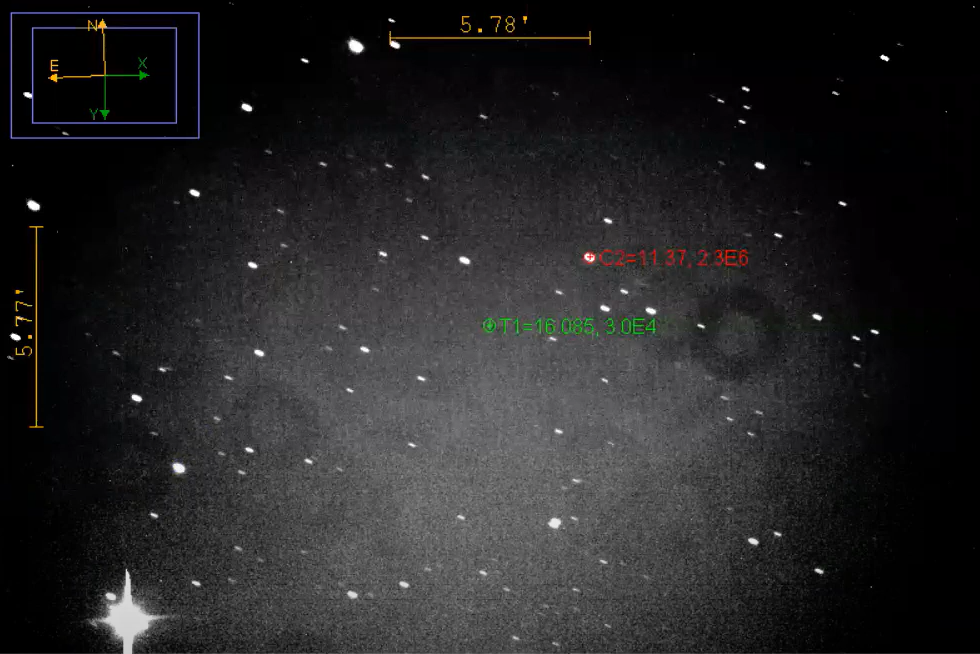
Lastly, a measurement log is generated which contains the specific RA and Dec of the stars, as seen in Figure 3.
Figure 3: A measurement log for the asteroid 6200. Generated using AstroImageJ.
Submission
Submitting the results to the Minor Planet Center requires a minimum data submission in a plain-text format [6]. It was required to have observed at least six numbered minor planets each on pairs of nearby nights as well as one numbered Near-Earth object observed on two distinct nights. It was also advised not to observe very low numbered objects or very bright objects, for this reason, all our studied asteroids were of magnitude 14-18. For new observing sites, the Minor Planet Center requires the astrometric accuracy of the observations to be within 2 arcseconds from the predicted ephemeris before designating an official observatory code for HCC. While arcseconds may sound like measurements of time, they are measurements of angle. It is formally known as a unit of angular measure equal to 1/60 of an arcminute and 1/3600 of a degree. Submission of this project will allow for future astrometric contributions to the Minor Planet Center.
Results
The team analyzed four asteroids using the data reduction methods listed previously and constructed a spreadsheet from the generate measurement logs to analyze the data [7]. Note that “Target” means what we calculated and “Predicted” is what we would consider the actual value – it is the value given by the MPC.
We used the mid-exposure time of our images to search for the actual coordinates of the asteroids through asteroid.lowell.edu and used the coordinates nearest in time for our spreadsheet [7]. Conversion from hours:minutes:seconds form and degrees:minutes:seconds form for RA and Dec, respectively, to hour decimal form was required for the coordinates received from asteroid.lowell.edu. This was to calculate the Δ arcseconds of RA and the Δ arcseconds of Dec. The respective conversion equations are below.
where hours, minutes, and seconds, represent the numbers in the corresponding sections in the hours:minutes:seconds form for RA.
where degrees, minutes, and seconds, represent the numbers in the corresponding sections in the degrees:minutes:seconds form for Dec.
To find the average total Δ arcseconds for each image, we used the equation below.
where in the above equation R stands for Δ arcseconds of RA, D stands for Δ arcseconds of Dec, and d stands for the predicted Dec converted into radians [8].
In the following graphs, the Δ arcseconds, as well as the Δ arcseconds of RA and the Δ arcseconds of Dec are visualized according the asteroid they were taken from.
|Δ arcsec in order| vs. asteroid number
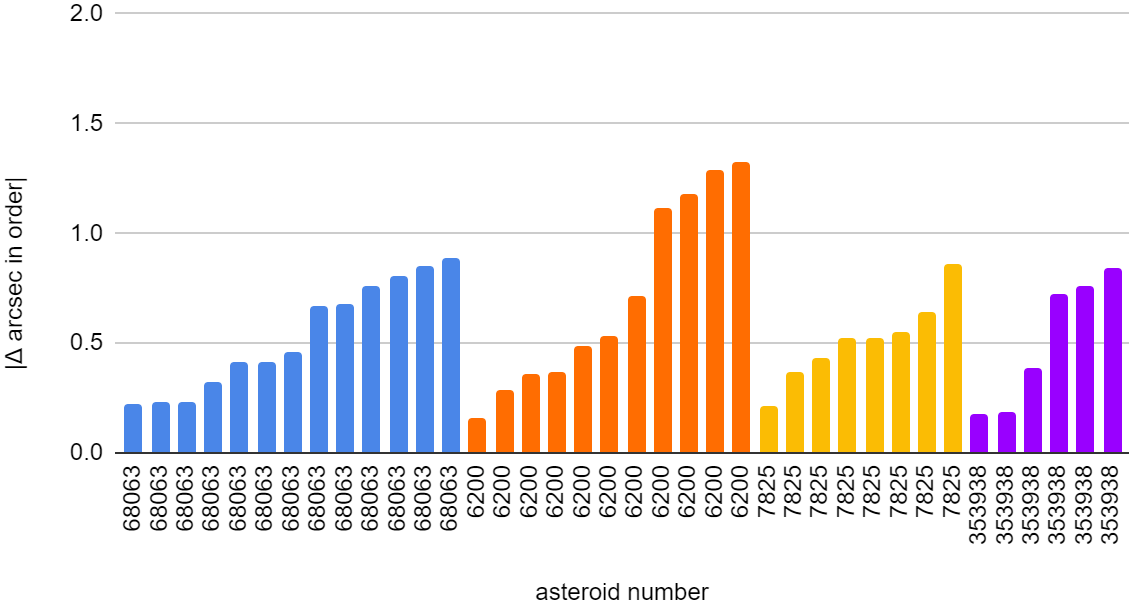
The average Δ arcseconds for each image are graphed and grouped according to their respective asteroid (Figure 4). Within each asteroid’s section on the graph, the Δ arcseconds are ordered by the magnitude of the error. Since the values fall below the MPC guidelines of 2.0 arcseconds or less error, this chart affirms that HCC can accurately track and predict asteroids’ positions.
|Δ arcsec ra in order| vs. asteroid number
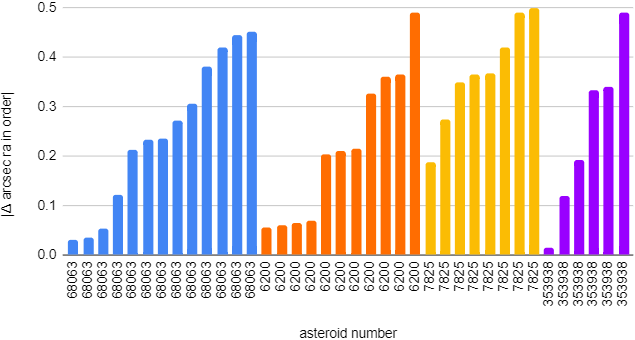
The average Δ arcseconds for ra for each image are graphed and grouped according to their respective asteroid (Figure 5). In each asteroid’s section, the Δ arcseconds for ra are ordered by the magnitude of error. Well within the MPC guidelines of 2.0 arcseconds or less error, this chart affirms that HCC can accurately track and predict asteroids’ ra.
|Δ arcsec dec in order| vs. asteroid number
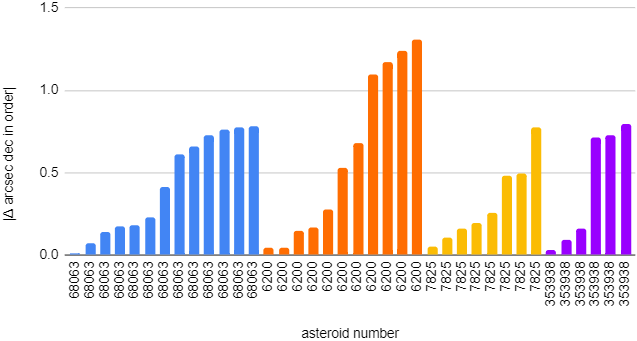
The average Δ arcseconds for dec for each image are graphed and grouped according to their respective asteroid (Figure 6). In each asteroid’s section, the Δ arcseconds for dec are ordered by the magnitude of error. Well within the MPC guidelines of 2.0 arcseconds or less error, this chart affirms that HCC can accurately track and predict asteroids’ dec. Note that the error with respect to dec is, at its max, 2.6 times greater than the error with respect to ra.
|Δ arcsec ra| and |Δ arcsec dec| vs. asteroid number
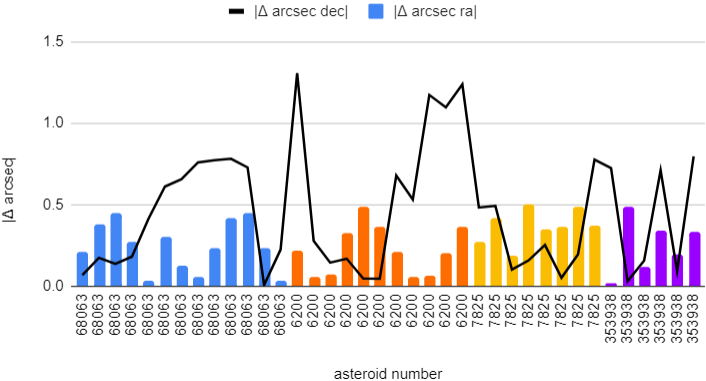
The average Δ arcseconds for ra for each image are graphed and grouped according to their respective asteroid (Figure 7). The average Δ arcseconds for dec for each image are also graphed and grouped according to their respective asteroid. Well within the MPC guidelines of 2.0 arcseconds or less error, this chart affirms that HCC can accurately track and predict asteroids’ ra and dec. No direct corellation between error in ra and error in dec is apparent from this graph.
|Δ mag| and average |Δ mag| vs. asteroid number
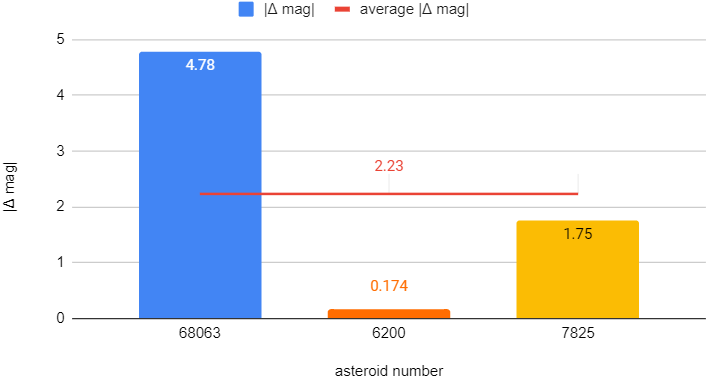
The error of each of our asteroids’ magnitude measurements are graphed using the formula for Δ arcseconds, with the line between the three representing the average error of our magnitude measurements (Figure 8). While MPC doesn’t require magnitude error to be below a certain amount in order to receive an observatory code [9], our varying data means we cannot accurately measure and predict an asteroid’s magnitude.
The team is confident that the four objects shown in this paper are asteroids and not just white spots on our images that are caused by cosmic rays. This is for two primary reasons. First, the white spots caused by cosmic rays are very small, just a few pixels in size. Our asteroids were several times this size. Second, white spots from cosmic rays are random. Meanwhile, we determined using star charts of the sections of sky our images were of that our asteroids followed a trajectory very close to the recorded trajectory for those asteroids.
Conclusion
From our results, it can be concluded that we are capable of measuring and determining position data, in terms of RA and DEC with an accuracy of <2 arcseconds. By nature of this, our research is substantial to the Minor Planet Center which in turn, allows us to contribute to the international database of asteroids.
In terms of questions left unanswered, the error presented in the Dec is twice that of RA. The reason for this is yet to be determined. Additionally, the estimation of the magnitude is imprecise and unreliable, with some targets being as much as 4.78 degrees of magnitude off, while others are comparatively closer to the expected value. In regards to this wide variabilty, a couple ideas could be suggested. For instance, optimization of the data reduction process could narrow down the wide variability. This would involve being more quantitatively selective about the choice in comparison stars, in terms of star radius and/or brightness; as opposed to the current method where comparison stars are selected arbitrarily, rather than numerical radii and brightness. This means selecting stars that are of similar radius to the target star and stars that are of similar magnitude to the target star.
Another concern worth exploring might be the radial pixel distance of the comparison stars to the target star. Perhaps comparison stars closer to the target star yield different results than comparison stars which are further away from the target star and each other. Comparison stars act as constants since their movement is so slow when compared to the asteroid in the image and the universe in general, that they appear to be standstill. Hence, movement of a comparison star is improbable and not a possible source of error.
Concerning future work, we propose attempting a light curve analysis of an asteroid [10]. Asteroids shine as sunlight reflects off their surface, hence the asteroids phase varies as it orbits around the sun. The greater the surface area of the asteroid that is exposed to sunlight, the brighter it appears and vice versa. Also, the distance of the asteroid to us affects its brightness, as closer objects appear brighter and farther objects appear dimmer. A light curve will especially be useful because, if the orbit of the asteroid is known, other confounding determinants, such as the asteroids distance from us, as well as the asteroid’s phase as it orbits around the sun, can be numerically calculated. Those factors can then be removed from the measured light curve; resulting in an light curve which is affected only by the spinning of the asteroid on an axis.
By refining the skills needed to track asteroids using a small telescope, we hope to achieve the precision and consistency needed to acquire an observatory code and carry out official follow-up observations for the Minor Planet Center. This is a pivotal step to allow for the possibility of future research seeking to contribute more knowledge to the field of astrophysics.
If you wish to access the data the authors collected, please contact them using the information provided below.
Contacts: earhavbarien@howardcc.edu, rericson@howardcc.edu, bdiamond@howardcc.edu
References
[1] Jimenez, E. Quintero and J. Aguirre, “ACQUISITION OF THE MINOR PLANET CENTER CODE FOR THE ASTRONOMICAL OBSERVATORY OF THE TECHNOLOGICAL UNIVERSITY OF PEREIRA (W63)”, TECCIENCIA, vol. 12, no. 23, pp. 43-50, 2017. Available: 10.18180/tecciencia.2017.23.6.
[2] “Main Page”, net, 2022. [Online]. Available: https://minorplanetcenter.net/. [Accessed: 06- Apr- 2022].
[3] “NASA – Amateur Astronomy”, gov, 2022. [Online]. Available: https://www.nasa.gov/vision/universe/watchtheskies/stars_hobby.html. [Accessed: 06- Apr- 2022].
[4] Hinchey, W. Jeffreys and P. Samadani, “Fantastic Exoplanets and Where to Find Them”, Journal of Research In Progress, vol. 2, pp. 1-11, 2019.
[5] Lang, D. Hogg, K. Mierle, M. Blanton and S. Roweis, “ASTROMETRY.NET: BLIND ASTROMETRIC CALIBRATION OF ARBITRARY ASTRONOMICAL IMAGES”, The Astronomical Journal, vol. 139, no. 5, pp. 1782-1800, 2010. Available: 10.1088/0004-6256/139/5/1782.
[6] net, 2022. [Online]. Available: https://minorplanetcenter.net/iau/info/obscode_application_submission. [Accessed: 06- Apr- 2022].
[7] Arhavbarien, R. A. Ericson, and B. Diamond, “Asteroid Observation Results Spreadsheet”, unpublished.
[8] Richmond, “Celestial Coordinates”, Web.archive.org, 2022. [Online]. Available: http://web.archive.org/web/20220128022423/http://spiff.rit.edu/classes/phys373/lectures/radec/radec.html. [Accessed: 06- Apr- 2022].
[9] “Guide to Minor Body Astrometry”, net, 2022. [Online]. Available: https://minorplanetcenter.net/iau/info/Astrometry.html. [Accessed: 06- Apr- 2022].
[10] “Light Curve Analysis”, NASA, 2022. [Online]. Available: https://www.nasa.gov/content/asteroid-grand-challenge/characterize/light-curve-analysis. [Accessed: 06- Apr- 2022].